What do the utility-scale quantum computers of the future look like? We believe that silicon spin-photon qubits are the path to utility-scale quantum applications and we are continuously innovating to extend their capabilities. We are excited to announce a new paper in collaboration with our partners at Simon Fraser University, “Electrically-triggered spin-photon devices in silicon”, now available on arXiv. This work introduces a new electrical control method for the silicon T centre, our qubit of choice. Electrical control is one of several methods to increase the number of qubits on a chip and entangle them faster.
In this research we show how T centres can be combined with diodes to create light-emitting diodes (LEDs) in the telecommunications O-band. By placing a single T centre in one of these LEDs, we show that we can produce single photons on demand with electrical pulses and couple them to an optical fibre. We also show that many T centres can be simultaneously excited in waveguides for use as an on-chip light source, producing hundreds of thousands of photons per second.
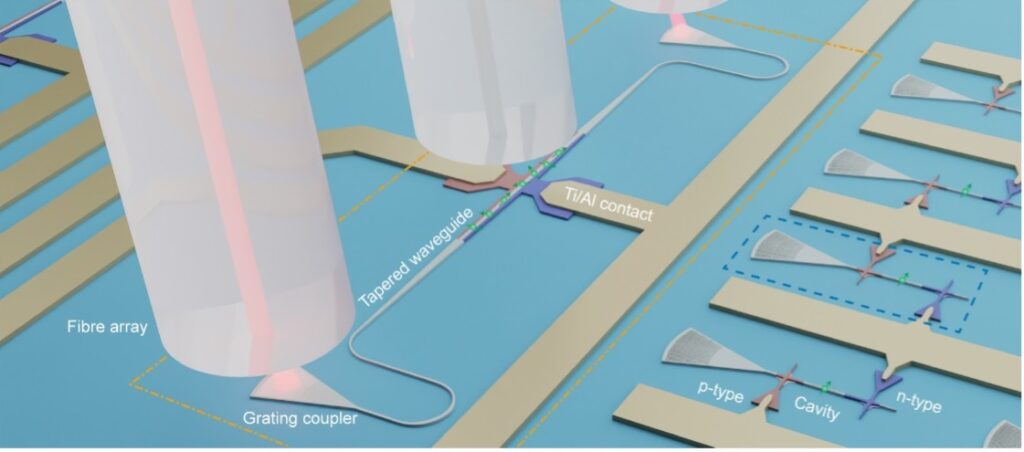
Illustration of a silicon chip with T centres in optoelectronic devices.
This work is the first demonstration of an electrically-injected single-photon source with silicon colour centres. Single photons in silicon photonics are the backbone of our quantum computing architecture, allowing us to distribute entanglement and control our qubit spin states. Our current architecture uses lasers to optically trigger the photon emission from T centres, but the devices in this new work add an additional electrical control mechanism. Not only do we show that photon emission from a single T centre can be triggered electrically, but we use this emission to initialize the spin qubit. To do this, we first electrically excite the T centre and filter its emission for a specific energy. By detecting a photon with a specific energy, we then know the electron spin state of the T centre. Once initialized, the T centre can be used for further quantum operations as usual.
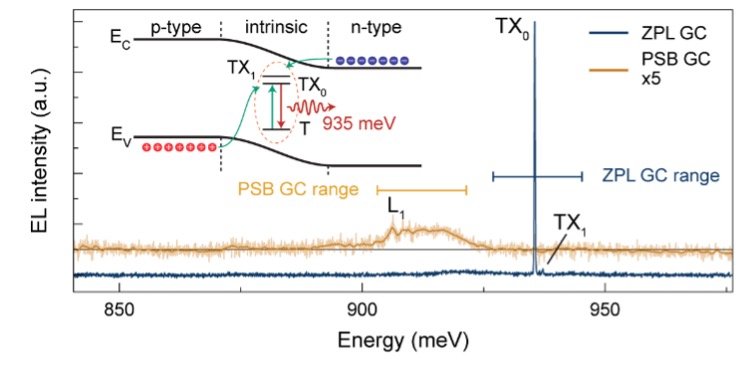
Electroluminescence spectrum of a T centre ensemble in a tapered waveguide device. The inset shows the luminescence mechanism in a diode under forward bias.
Single photons are desired for many other quantum technologies including all-optical quantum computers and quantum networks. Such a wide range of applications is made possible by the T centre’s unique advantages: combining telecommunications-band optical emission, long-lived spin qubits and silicon integration. In silicon it is possible to combine the photonic and electronic elements required for these devices. A silicon single-photon source can be networked on-chip with a wide range of well-established silicon photonic devices including detectors, switches and high-quality optical resonators. Previous electrically-triggered photon sources for silicon photonics relied on challenging and costly hybrid material processing.
What does this mean for future quantum technologies? We are always looking for new and innovative ways to increase the number of qubits on a chip, and their performance. Rather than routing laser pulses to every T centre, these new devices could be used to trigger entanglement electrically between many T centres simultaneously. In the future, this technology could also be used to entangle spin qubits. This approach may reduce the resources required for large-scale quantum computers. While optical controls will still be needed for some tasks, this parallel electrical control has the potential to increase the number of qubits that can fit on a chip and entangle them faster.
Key takeaways:
- Single silicon T centres can be electrically excited, for single-photon sources directly integrated with silicon photonics.
- Electrical initialization of the T centre spin qubit enables a new parallelizable control mechanism.
- Future schemes that leverage electrical control may allow more qubits to fit on a chip, as well as faster entanglement.
We would like to acknowledge the contributions of all involved in this research.